This review discusses the increasing application of nanomaterials in ion-selective electrodes for public health, emphasizing their role in healthcare, pharmaceuticals, and the food industry. It addresses the use and challenges of carbon-based nanoparticles in wearable devices for better patient monitoring and preventative healthcare. Additionally, it contrasts these electrodes with traditional medical methods, highlighting their cost efficiency, accessibility, and real-time capabilities. The review also looks forward to the evolution of sensor technology and nanoparticle research, underlining the importance of interdisciplinary collaboration to advance health monitoring by integrating nanomaterials with ion-selective electrodes.
Author ID: 2024024866
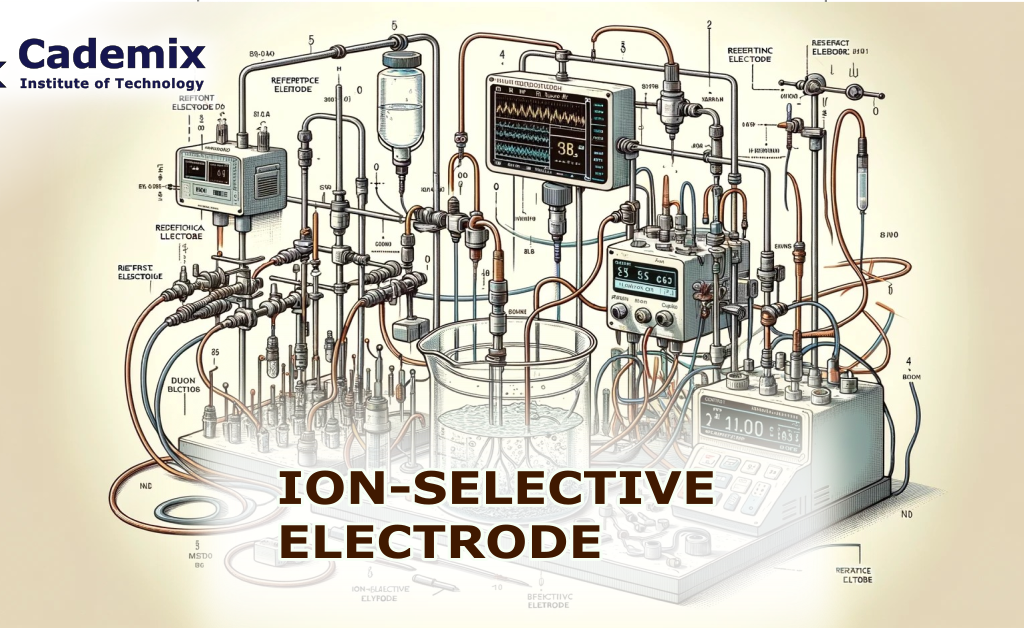
1. Fundamentals of Wearable Ion-Selective Electrodes
Wearable ion-selective electrodes represent a groundbreaking advancement in health monitoring technologies. At their core, these devices utilize the principles of ion-selective electrodes (ISEs), traditionally used in laboratory settings to measure ion concentrations in various solutions precisely. It is significant that the transition of this technology into a wearable format opened new horizons in personal healthcare, enabling continuous, real-time monitoring of physiological parameters.
The basis of wearable ion-selective electrodes lies in their ability to selectively respond to specific ions in the body [1]. This sensitivity comes from a special membrane in the electrode that reacts mostly to certain types of ions. When it touches a liquid like sweat or blood, the electrode’s potential changes based on how much of the specific ion is present. This electrochemical reaction forms the foundation for ion concentration analysis, providing crucial data on the wearer’s health status. Furthermore, an ion-selective electrode is considered the most important device in the food industry to regulate the level of ions to prevent illnesses.
Integrating nanomaterials plays a crucial role in enhancing the performance of these electrodes. Nanoparticles, particularly carbon-based materials like graphene, carbon nanotubes, and fullerene, revolutionized the sensitivity and selectivity of these devices. Their unique properties, such as high surface area, excellent conductivity, and chemical stability, make them ideal for biosensing applications. The devices achieve higher sensitivity, faster response times, and greater miniaturization by incorporating these nanomaterials into the electrode’s sensitive membrane, which is essential for wearable technology.
In wearable applications, the ion-selective electrodes are typically part of a more extensive system that includes a signal processing unit and a wireless transmitter. This system collects and analyzes the electrode’s data, translating the electrochemical signals into understandable health metrics. The data could be transmitted to a smartphone or a remote healthcare provider, enabling quick medical help.
However, developing these electrodes for wearable use is not without challenges. Ensuring long-term stability, maintaining consistent performance in varying environmental conditions, and minimizing interference from other bodily substances are ongoing research areas. Integrating these sensors into comfortable, user-friendly wearable formats requires innovative engineering and design solutions.
Incorporating nanomaterials enhances wearable ion-selective electrodes and significantly advances health monitoring technology. They offer the promise of personalized health care, with potential applications ranging from fitness tracking to early detection and management of medical conditions. As this technology continues to evolve, it holds immense potential for transforming healthcare by providing insights into our body’s ionic composition in real-time, paving the way for more proactive and preventive health management strategies.
2. Comparative ion-selective electrode with Traditional Medical Techniques
In this comparison, we look at the benefits of cost-effective ion-selective electrodes over traditional methods for monitoring various ions in the body, environment, and food safety. Conventional techniques such as titrations, spectroscopy, etc., are often expensive and complex, involving lab analyses requiring special equipment and trained staff. While accurate, they can be less accessible and slower, making real-time monitoring and quick decisions difficult.
In contrast, the introduction of affordable ion-selective electrodes is a significant improvement. They are budget-friendly and easy to use, making monitoring more available to a broader audience, not just specialized labs Table 1. This is especially important in places with limited resources, where traditional methods might not work. Additionally, these electrodes allow for real-time monitoring, enabling faster reactions to changes in ion levels. This is crucial in areas like agriculture, healthcare, and the food industry to control the level of ions to prevent illnesses.
While these electrodes are cost-effective and user-friendly, it’s essential to evaluate their accuracy and reliability against traditional methods. Ongoing improvements in electrode technology are focused on closing any gaps in precision. The goal is for these tools to be practical, affordable, and accurate in ion-level monitoring. Therefore, these electrodes are a promising advancement in ion solution analysis, offering efficiency, affordability, and the potential for broad application.
Table 1. Comparison of ion-selective electrodes with traditional approaches
Method | Advantages | Disadvantages |
---|---|---|
Spectrophotometry | – Relatively inexpensive equipment. – Good for quantitative analysis of specific compounds. – Broad application in organic and inorganic chemistry. | – Reagents and maintenance add to costs. – Limited by the specificity of the reagents. – Generally not portable. |
Atomic Absorption Spectroscopy (AAS) | – High sensitivity for metals and some non-metals. – Cost-effective for specific ion measurements. – Durable, long-lasting equipment. | – High initial equipment cost. – Limited to elements that can be atomized. – Requires specific expertise and training. |
Inductively Coupled Plasma Mass Spectrometry (ICP-MS) | – Very high initial equipment cost. – Large, complex systems, not portable. – Intensive maintenance and calibration are needed. | – Very high initial equipment cost. – Large, complex systems, not portable. – Intensive maintenance and calibration needed. |
Titrations | – Simple and straightforward method. – No need for expensive equipment. – High accuracy for concentration determination. | – Time-consuming, especially for multiple samples. – Requires skilled technique for accurate results. – Limited to the titrant’s reactive properties. |
Ion-Selective Electrodes (ISEs) | – Initial development can be expensive. – Some analyses may require longer processing times. – Regular calibration and maintenance are needed. | – Initial development can be expensive. – Some analyses may require longer processing times. – Regular calibration and maintenance needed. |
3. Nanoparticles in Wearable Health Devices
In the world of electrochemical sensors, particularly for health tracking, nanomaterials have sparked a surge of innovation. Right now, the focus is mainly on carbon-based nanomaterials that are crucial for boosting the performance of wearable electrodes.
3.1. Carbon-Based Nanomaterials for Electrodes
In developing electrochemical sensors for health monitoring, carbon-based nanomaterials emerged as key elements, offering distinct advantages in electrode design. These materials, particularly graphene and carbon nanotubes, are highly valued for their unique electrical and mechanical properties.
Graphene, characterized by its two-dimensional structure of a single layer of carbon atoms, provides exceptional electrical conductivity and a large surface area. These attributes are critical in enhancing the sensitivity and responsiveness of ion-selective electrodes, making them more efficient for wearable health monitoring devices. The ability of graphene to detect subtle changes in ion concentrations swiftly makes it a valuable component in advanced health monitoring technologies.
Carbon nanotubes, an essential type of carbon-based nanomaterial, add strength and chemical steadiness to electrodes. Their tube-like nanostructure provides a sizeable reactive surface, which is crucial for accurate and selective ion detection. The sturdiness of carbon nanotubes helps wearable sensors last longer, which is vital for regular health tracking. Using these carbon-based materials in wearable electrodes is a big step forward in sensor technology, improving both how well health monitors work and how easy they are to use.
3.2. Innovations in Nanoparticle Integration
In the rapidly advancing field of wearable electrochemical sensors, adding nanoparticles is a significant breakthrough. This advancement is not just in the materials themselves but also in the innovative ways they are incorporated into sensor designs.
Recent developments seen a surge in creative techniques for embedding nanoparticles into wearable electrodes. One approach involves creating composite materials that blend nanoparticles with flexible, biocompatible substrates. This method ensures that the sensors are highly sensitive due to the nanoparticles and are comfortable and practical for everyday wear. The development of more user-friendly yet still-effective wearable health monitoring is revolutionized by using these composites.
Additionally, advancements in nanofabrication techniques allow for more precise placement and orientation of nanoparticles within the sensors. This precision is crucial in enhancing the selectivity and sensitivity of the electrodes, ensuring that they can accurately detect specific ion concentrations in real time. Combining nanoparticles with these sophisticated methods is crucial in expanding the capabilities of wearable health monitoring devices, leading to more precise, dependable, and user-friendly health tracking options.
3.3. Challenges in Nanomaterial Application
In the field of wearable electrochemical sensors, the application of nanomaterials, while pioneering, presents its own challenges. These obstacles must be navigated to fully harness the potential of these advanced materials in health monitoring devices.
One significant challenge in the application of nanomaterials is the manufacturing process. Creating electrodes incorporating nanomaterials like graphene and carbon nanotubes requires precision and consistency. The process must be scalable and cost-effective for widespread adoption, which is a complex endeavor. Ensuring the uniformity and quality of nanomaterials across large batches is crucial for the reliability of wearable sensors. This aspect of manufacturing remains a focus area for ongoing research and development.
Another critical issue is the stability and durability of nanomaterials in varying environmental conditions. Wearable sensors expose the variation of temperatures, humidity, and physical stresses, which can impact the performance of nanomaterials. It’s important to ensure these materials keep their sensitivity and effectiveness over time, particularly in the bendable forms needed for wearable technology. Recently, researchers explore ways to enhance the stability of nanomaterials, aiming to extend the lifespan and effectiveness of wearable ion-selective electrodes in everyday use.
4. Patient Monitoring and Disease Prevention
In the changing world of healthcare, “Patient Monitoring and Disease Prevention” emerge as a crucial area. Wearable ion-selective electrodes are making a significant impact here. Using advanced nanomaterials, these devices allow for real-time health monitoring and offer ongoing tracking of vital health signs.
4.1. Real-Time Health Monitoring
Introducing wearable electrodes revolutionizes health monitoring by moving from occasional checks to continuous tracking. These devices, equipped with advanced ion-selective electrodes, provide real-time health monitoring, a key development for proactive healthcare management.
Wearable electrodes constantly monitor crucial health indicators, offering a continuous flow of data that is extremely useful for overseeing and managing chronic illnesses. This instant data gathering lets us quickly notice changes in the body, leading to fast medical responses. For people with conditions like diabetes or heart disease, this ongoing monitoring can be lifesaving. It provides insights into their health that were not possible with sporadic testing approaches.
Furthermore, the convenience and non-invasiveness of these wearable devices encourage consistent usage, ensuring continuous health data collection. This aspect is crucial in building comprehensive health profiles, aiding in early disease detection and preventive healthcare. Wearable electrodes provide continuous health monitoring in an easy-to-use format, establish new benchmarks in patient care, and integrate health tracking smoothly into everyday life.
5. Application of Ion-selective electrodes in the Healthcare system
Maintaining balanced ion levels in the body is essential for preventing various diseases [2]. Ions such as potassium, sodium, calcium, magnesium, etc., play crucial roles in vital bodily functions, including regulating nerve impulses, muscle contractions, and hydration levels. Imbalances can lead to conditions like hypertension due to excess sodium or hypokalemia from low potassium levels, which can affect heart and muscle function. Calcium and magnesium are vital for bone health, and their deficiency can lead to osteoporosis. Regular monitoring and managing ion intake through diet or medication can help maintain these levels, ensuring health and preventing disease onset .
At Metrohm Company, the researchers designed a method for measuring potassium levels using an ion-selective electrode (ISE). This method involves a specific electrode sensitive to potassium ions, allowing for the accurate and selective measurement of potassium concentrations in various samples. The article’s focus on ion-selective electrode technology underscores its importance in analytical chemistry and diagnostics, as well as potential applications of this technique in fields like healthcare, environmental monitoring, or research [3].
BIOS Lab-on-a-Chip Group focuses on developing and using solid contact potassium selective electrodes in various biomedical contexts. They explore ion-selective electrodes and their applications in the medical and biological sciences and biomedical applications [4].
Odijk et al. designed a novel ion-selective to measure the level of potassium in the brain, which is crucial in understanding brain disorders[5].
At Heinrich Heine University Düsseldorf, Rose et al. designed microelectrodes to measure extracellular ion concentrations, particularly in brain tissues, and they are essential for understanding the ionic dynamics during various neural activities. The double-barreled and concentric designs of these microelectrodes allow for precise and simultaneous measurement of different ions or the combination of ionic measurement with other physiological parameters. This research is vital for advancing our knowledge of brain function and the ionic mechanisms that underlie neural activity and disorders [6].
Miras et al. developed cost-effective ion-selective electrodes for monitoring potassium and nitrate levels in nutrient solutions. These electrodes are particularly valuable in agriculture and hydroponics, where nutrient management is crucial for optimal plant growth. The study probably explores the design and fabrication of these electrodes, emphasizing their affordability and efficiency in simultaneously measuring multiple ions. This approach could significantly improve nutrient management by providing real-time, accurate data on crucial nutrient levels, enabling more precise control over the nutrient environment. The article would be particularly relevant for researchers and practitioners in plant science, agriculture, and environmental monitoring, offering insights into advancing technology in nutrient analysis while maintaining cost-effectiveness [7].
At Åbo Akademi University, researchers developed the new potassium ion-selective electrodes (ISEs) using lipophilic multi-walled carbon nanotubes (MWCNTs) as the solid contact. These electrodes indicated high sensitivity and selectivity towards potassium ions, which are essential in various scientific and environmental applications. The focus is on leveraging the unique properties of these electrodes for precise and efficient analysis in the field of analytical chemistry [8].
Isildak et al. designed a novel membrane electrode to determine nitrate ions in water samples and fruNANit juices. It focuses on using membrane electrodes infused with silver bis diethyldithiocarbamate for their high selectivity and sensitivity to nitrate ions. This potentiometric approach, known for its simplicity and cost-effectiveness, offers precise measurements with minimal interference from other substances. However, challenges might include the membrane’s potential instability and the method’s specificity to nitrate ions, which could limit broader application. Overall, the study contributes significantly to advancements in ion-selective electrode technology, enhancing nitrate ion detection in various environments [9].
designed the novel ion-selective electrodes based on multiwall carbon nanotubes to measure the level of salicylate ions in the Aspirin table, which could prevent some diseases [10].
6. Risks, Challenges, and Ethical Considerations
6.1. Risk Assessment in Wearable Sensing
This section delves into the potential risks associated with using wearable tech devices, emphasizing the need for thorough evaluation and management of these risks. Wearable technologies, rapidly becoming integral in healthcare for monitoring and diagnostic purposes, bring unique challenges in terms of privacy, security, and health-related risks. The primary concern revolves around the security and privacy of the data collected by these devices. Sensitive health information, if not adequately protected, can be vulnerable to breaches, posing significant privacy threats. Furthermore, the accuracy and reliability of these devices in health monitoring are crucial. Any inaccuracies in data could lead to misdiagnosis or inappropriate health interventions, potentially endangering users’ health.
Additionally, the long-term health impacts of continuously wearing these devices, including potential skin irritations, allergies, or other side effects, require thorough investigation. As wearable technologies become more prevalent, it’s essential to balance their benefits with a comprehensive understanding and mitigation of these risks. This involves rigorous testing and standards compliance and clear communication with users about potential risks and safe usage guidelines. While wearable technologies hold immense potential in healthcare, a careful and informed approach is necessary to maximize their benefits while safeguarding against the associated risks.
6.2. Overcoming Technical Challenges
This section discussed the challenges faced in developing and applying advanced technologies. A key obstacle is integrating new systems into existing setups. This requires careful planning and design to ensure compatibility and smooth functioning. As technologies evolve, they often necessitate new protocols and standards for effective and safe operation. This adaptation isn’t just technical; it also involves educating and training users to use these technologies effectively.
Moreover, the dependability and longevity of new technologies are critical, especially in essential applications. Maintaining consistent performance in various conditions and over long periods is challenging. This demands thorough testing and ongoing enhancements based on feedback and performance data. In tackling these technical hurdles, the focus should not only be on the technological side but also the user experience. It’s vital to ensure the technology is advanced, easy to use, and accessible. Directly addressing these challenges is essential for successfully implementing and broadly adopting new technologies.
7. Future Perspective and Interdisciplinary Collaboration
Emerging trends in sensor technology are shaping the future of wearable health devices, making them more sophisticated and effective. These advancements are especially notable in integrating advanced wearable sensors, revolutionizing personal health monitoring and management. Ion-selective electrodes, particularly those utilizing nanomaterials, are at the forefront of these developments. However, they face challenges in maintaining reliability over time and in various environments, which is crucial for accurate health tracking. Another challenge is designing these high-tech devices to be user-friendly and comfortable for everyday wear. As the technology evolves, there is a growing need for electrodes capable of monitoring a broader range of ions and health indicators, enhancing their utility in healthcare.
Progress in creating more effective wearable ion-selective electrodes with nanomaterials demands collaboration across multiple disciplines, including materials science, biomedical engineering, data analysis, and healthcare. The focus is shifting towards improving these devices’ functionality and user experience to meet diverse health monitoring needs. Additionally, addressing ethical concerns such as data privacy and security is paramount. Collaborative efforts across these fields are essential in overcoming these challenges and fully realizing the potential of this technology to transform health monitoring and disease prevention.
8. References
[1] Heikenfeld, J., Jajack, A., Rogers, J., Gutruf, P., Tian, L., Pan, T., Li, R., Khine, M., Kim, J. and Wang, J., 2018. Wearable sensors: modalities, challenges, and prospects. Lab on a Chip, 18(2), pp.217-248. DOI: 10.1039/c7lc00914c [2] Dimeski, G., Badrick, T. and St John, A., 2010. Ion-selective electrodes (ISEs) and interferences- a review. Clinica Chimica Acta, 411(5-6), pp.309-317. DOI: 10.1016/j.cca.2009.12.005 [3] https://www.metrohm.com/ [4] https://www.utwente.nl/en/eemcs/bios/ [5] Odijk, M., Van Der Wouden, E.J., Olthuis, W., Ferrari, M.D., Tolner, E.A., Van Den Maagdenberg, A.M.J.M. and van den Berg, A., 2015. Microfabricated solid-state ion-selective electrode probe for measuring potassium in the living rodent brain: Compatibility with DC-EEG recordings to study spreading depression. Sensors and Actuators B: Chemical, 207, pp.945-953. DOI:10.1016/j.snb.2014.06.138 [6] Haack, N., Durry, S., Kafitz, K.W., Chesler, M. and Rose, C.R., 2015. Double-barreled and concentric microelectrodes for measurement of extracellular ion signals in brain tissue. JoVE (Journal of Visualized Experiments), (103), p.e53058. DOI: 10.3791/53058 [7] Miras, M., García, M.S., Martínez, V. and Ortuño, J.Á., 2021. Inexpensive ion-selective electrodes for the simultaneous monitoring of potassium and nitrate concentrations in nutrient solutions. Analytical Methods, 13(31), pp.3511-3520. DOI: 10.1039/D1AY00956G [8] Papp, S., Kozma, J., Lindfors, T. and Gyurcsányi, R.E., 2020. Lipophilic Multi‐walled Carbon Nanotube‐based Solid Contact Potassium Ion‐selective Electrodes with Reproducible Standard Potentials. A Comparative Study. Electroanalysis, 32(4), pp.867-873. DOI: 10.1002/elan.202000045 [9] Isildak, O. and Yildiz, I., 2023. Highly selective potentiometric determination of nitrate ions using silver bisdiethyldithiocarbamate based membrane electrodes. Electrochimica Acta, 459, p.142587.DOI: 10.1016/j.electacta.2023.142587 [10] Jahromi, Z.A.Y., Mazloum-Ardakani, M. and Reza, H., 2017. Using Potentiometry and Electrochemical Impedance Spectroscopy Techniques for Studying Effect of Nanomaterials on Salicylate Ion-selective Electrode. ANALYTICAL & BIOANALYTICAL ELECTROCHEMISTRY, 9(5), pp.562-573.