This article discusses the recent progress in electrochemical biosensors and their significant impact on point-of-care diagnostics, particularly for detecting microorganisms and aiding public health. It covers technological advancements in aptamers, phages, antibodies, and DNA biosensors and how nanomaterials improve the performance of biosensors. The article also addresses the challenges and solutions in this field, including ethical issues and risk management. It emphasizes the importance and growing use of these biosensors in various sectors, including food safety and medical diagnostics.
Author ID: 2024024866
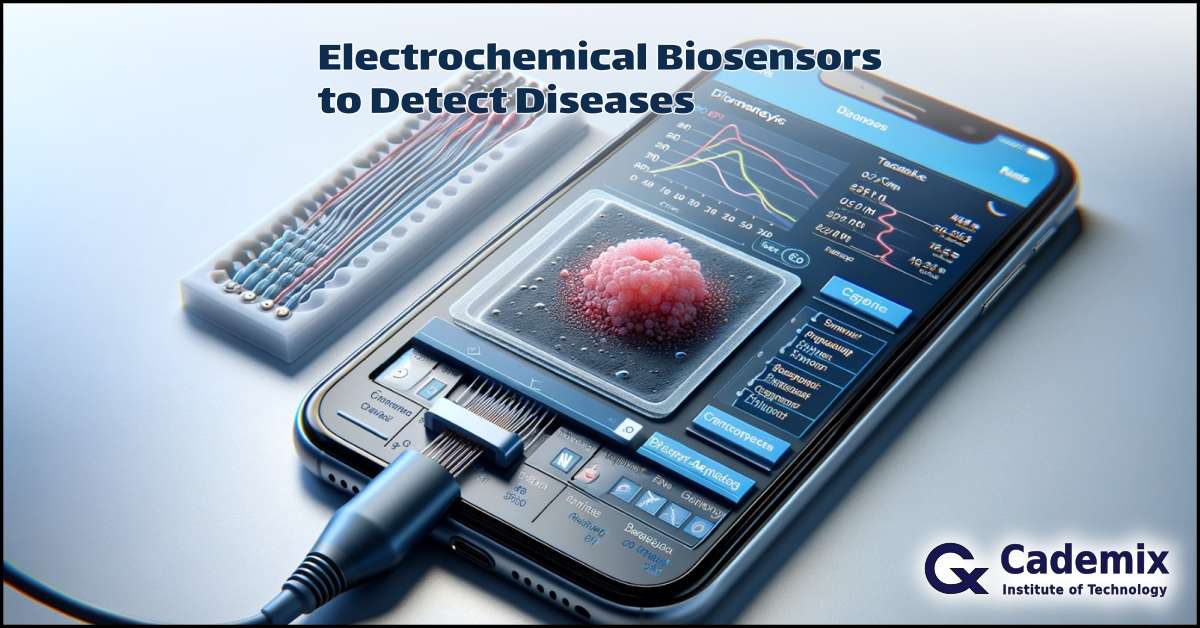
1. Electrochemical Biosensors in Microorganism Detection
The growing concern over foodborne pathogens in the food industry has become more pronounced in recent years. These harmful microorganisms in food pose significant risks to public health and can lead to substantial financial losses during food processing. Ensuring that food remains safe and high-quality is a formidable challenge in this sector. Therefore, rapidly and accurately detecting harmful bacteria in food products is paramount. Traditional methods for detecting these pathogens are often slow, require multiple steps, and expensive.
However, the advent of biosensors emerges as a highly effective solution for precisely detecting foodborne pathogens. A key advantage of biosensors is their ability to sensitively identify pathogens without the need for extensive sample preparation. These systems typically comprise three main components: transducers, which convert biological interactions into measurable signals such as electrochemical or optical signals; bioreceptors, including phage, aptamer, antibody and DNA, which are responsible for recognizing and binding to the target pathogens; and detectors, which record and quantify the signals [1].
electrochemical biosensing gain popularity as it offers rapid response times, affordability, high sensitivity, and stability. Additionally, nanomaterials become increasingly important in the fabrication of electrochemical nano-biosensors. Their use enhances electrical conductivity and facilitates the proper orientation of biorecognition elements, thereby improving the efficiency of bacterial capture. These advancements in biosensor technology are revolutionizing the detection of foodborne pathogens, offering faster, more cost-effective, and more accurate methods to ensure food safety.
2. Biorecognition elements
2.1. DNA aptamers
2.1.1.Emerging Trends: Aptamer Modification Techniques
The landscape of electrochemical biosensor design is rapidly evolving, with a significant focus on aptamer modification techniques. These advancements have led to the development of innovative DNA aptasensors that revolutionize point-of-care diagnostics. Researchers enhance the sensitivity and specificity of these biosensors by altering the structure and functionality of aptamers – specialized DNA or RNA molecules. This progress is particularly impactful in medical diagnostics, where the ability to detect minute amounts of biomarkers can mean the difference between early and late disease detection.
2.1.2. Applications aptasensor to identify pathogenic bacteria
Aptamers in electrochemical biosensors are versatile, serving food quality assessment and biomedical diagnostics. Their capacity to selectively attach to various targets, from foodborne pathogens to cancer biomarkers, makes aptasensors invaluable in ensuring food safety and detecting diseases early. They shine across diverse sectors. Creative Biolabs focuses on the applied electrochemical aptamer-based biosensors for broad research applications in biomedical diagnostics [2].
E.Ferapontova et al. designed a new, cost-effective immunomagnetic assay that detects single bacterial cells, such as Escherichia coli, with high specificity and sensitivity. This method uses a combination of cellulase enzymes and either aptamers or antibodies on magnetic beads to alter an electrode’s electrical conductivity, enabling the detection and quantification of bacteria without interference from other species. It is quick and adaptable, suitable for direct application in various environments such as clinical, food, and environmental samples [3].
Z.Yousefniayejahromi et al. designed the novel aptasensor using a screen-printed gold electrode to recognize E. coli bacteria in different biological samples, such as urine, feces, milk, and water [4].
2.1.3. Advances in Aptamer Selection and Modification
It is significant that selecting and modifying aptamers leads to progress in electrochemical biosensors. Systematic evolution of ligands by exponential enrichment (SELEX) is one technology that has been refined to produce aptamers with improved binding capabilities. These developments serve as the foundation for creating accurate, effective biosensors for various biological and chemical targets.
2.1.4. DNA Biosensors and Aptasensors: A Powerful Combination
Recently, combination of other bioreceptors with aptasensors is a noteworthy development in biosensor applications. This combination opens up a new era of biosensors by utilizing the selectivity of DNA and the versatility of aptamer-based detection. The development of quicker, more accurate point-of-care diagnostic tests is greatly aided by this technological pair, which is essential for rapid results
2.1.5. Future of Rapid Diagnostics: Aptamer-Integrated Electrochemical Biosensors
electrochemical aptasensors are critical to the future of quick diagnostic testing. With its quicker, more trustworthy, and more approachable testing options, this integration has the potential to revolutionize point-of-care diagnostics. Beyond their present uses, aptamer-based electrochemical biosensors have great potential for the future of personalized medicine, environmental monitoring, and other fields.
2.2. Bacteriophage
2.2.1. Introduction to Bacteriophage-Based Electrochemical Biosensors
Bacteriophages, or phages, are viruses that infect bacteria [5]. They gain attention in developing electrochemical biosensors due to their specificity in targeting certain bacterial strains. These biosensors detect the interaction between phages and their target bacteria, generating an electrochemical signal. The specificity of phages ensures that these sensors can precisely identify particular pathogens, making them ideal for detecting infectious diseases.
2.2.2. Working Principle of Phage-Based Biosensors
The core principle of phage-based electrochemical biosensors lies in the unique interaction between a bacteriophage and its specific bacterial host. When a bacteriophage binds to its target bacteria, it triggers a change in the electrochemical properties of the system. This change measures current, voltage, or impedance, which can be correlated to the presence and concentration of the target pathogen. Advanced techniques like amperometry, potentiometry, and impedance spectroscopy are commonly used to quantify these electrochemical signals.
2.2.3. Advantages in Infectious Disease Detection
In the context of infectious diseases, these biosensors offer several advantages. Their high specificity reduces the likelihood of false positives, a common challenge in pathogen detection. Additionally, electrochemical biosensors are known for their rapid response time, often delivering results in a matter of minutes. This is crucial in clinical settings where timely diagnosis can significantly impact patient outcomes. Moreover, these biosensors are typically portable and can be used in field settings, extending their applicability beyond traditional laboratory environments.
2.2.4. Recent Advances and Applications
Recent advancements in phage-based biosensors focus on enhancing their sensitivity and range of detectable pathogens. This includes engineering phages with increased binding affinity and integrating nanomaterials to amplify the electrochemical signal. Such developments broaden the scope of these biosensors, allowing for the detection of a wider range of infectious agents, including drug-resistant strains. Their application extends to various sectors, including healthcare, food safety, and environmental monitoring.
Z.Yousefniayejahromi et al. developed the Zeno phage sensor to identify Staphylococcus aureus in synovial fluid with a linear range from 101 to 104 CFU/ml with a detection limit of 6 CFU/mL [6].
Moghtader et al. enhanced the sensitivity of impedance spectroscopy by using pencil graphite electrodes coated with gold nanorods (GNRs). This approach significantly improved surface conductance. They immobilized an increased number of T4 phages onto these GNR-coated electrodes, aiming to detect
E. coli K12 more effectively. The study achieved a notable detection limit of 100 CFU/mL for identifying this target bacteria, showcasing the potential of this method in bacterial detection and biosensor technology [7].
2.3. Antibody
2.3.1. Introduction to Antibody-Based Electrochemical Biosensors
Antibodies are crucial components in the field of electrochemical biosensors, offering high levels of specificity and sensitivity for detecting various biomolecules. In these biosensors, antibodies serve as recognition elements that selectively bind to their corresponding antigens, which could be proteins, hormones, or pathogens. This selective binding is fundamental in detecting and diagnosing diseases, as it allows for the precise identification of specific biomarkers related to different health conditions. These biosensors convert the biochemical reaction between the antibody and antigen into an electrical signal, enabling quantifiable and rapid detection.
2.3.2. Advantages and Applications in Bactria Detection
The primary advantage of antibody-based electrochemical biosensors lies in their remarkable specificity and sensitivity. This makes them exceptionally useful in medical diagnostics, particularly for early detection of diseases. For example, they can detect minute amounts of cancer markers or viruses in a patient’s blood, leading to early intervention and treatment. Moreover, these biosensors are often designed to be portable and user-friendly, allowing for point-of-care testing. This means they can be used in various settings, from hospitals to remote areas, facilitating widespread access to critical diagnostic tools.
2.3.3. Challenges and Future Developments
While antibody-based electrochemical biosensors hold great promise, they also face challenges, mainly related to the stability and durability of antibodies. In certain conditions, antibodies can lose effectiveness, leading to inaccurate readings. Addressing this, current research focuses on enhancing antibody stability and integrating advanced materials to improve sensor performance. Future developments are likely to see the creation of more robust biosensors capable of functioning effectively in a wider range of environments and conditions. Additionally, integrating digital technologies and artificial intelligence is expected further to refine the accuracy and usability of these biosensors, paving the way for more personalized and efficient healthcare solutions.
2.4. DNA
2.4.1. Introduction to DNA Biosensors in Clinical Diagnostics
DNA biosensors are a groundbreaking technology in the field of clinical diagnostics. They are based on specific DNA sequences that are diagnostic of a range of medical illnesses, from infectious infections to genetic diseases. The core principle of these biosensors is their ability to bind selectively to target DNA sequences, allowing for identifying particular genetic markers. This specificity is crucial in medical diagnostics, as it enables healthcare professionals to diagnose conditions accurately and rapidly, which is essential for effective treatment. Ace Infectious developed the DNA-based nanosensors to recognize specific bacteria [8].
2.4.2. Challenges and Limitations
Despite the significant progress, DNA biosensors face challenges that need addressing to maximize their clinical utility. One of the primary challenges is ensuring the accuracy and reliability of the results, especially in the presence of complex biological samples. There is also the need to continuously improve the sensitivity of these sensors to detect very low concentrations of DNA. Furthermore, integrating these biosensors into existing healthcare systems poses logistical and economic challenges that must be considered for widespread adoption.
2.4.3. Advancements in DNA Biosensor Technology
Recent technological advances leads to boost the sensitivity and speed of DNA biosensors significantly. Innovations include the development of more sophisticated detection methods, such as electrochemical approaches. These methods improve the ability of DNA biosensors to detect low levels of genetic material, making them highly effective even in the early stages of disease. Additionally, advancements in miniaturization and automation cause the creation of portable biosensors, allowing for point-of-care testing and immediate results.
2.4.4. Clinical Applications of DNA Biosensors
Recently, clinical settings used DNA biosensors for various applications. They are particularly valuable in the early detection of cancers, where they can identify genetic mutations associated with different types of cancer. Similarly, in infectious disease management, these biosensors applied to detect pathogens’ DNA, enabling rapid diagnosis and the timely administration of appropriate treatments. This rapid detection is crucial for patient care and controlling the spread of infectious diseases, especially in outbreak scenarios.
2.4.5. Future Perspectives in DNA Biosensor Development
Looking ahead, the future of DNA biosensors in clinical diagnostics is promising. Ongoing research focuses on integrating advanced materials, like graphene and quantum dots, to enhance sensitivity and specificity further. Artificial intelligence and machine learning are also being explored to analyze complex data sets, which can lead to more precise diagnostics and personalized treatment plans. As these technologies evolve, DNA biosensors become integral to modern healthcare, offering rapid, accurate, and accessible diagnostics.
3. Comparision between different biorecognition elements
The biorecognition elements, such as antibodies, aptamers, bacteriophages, and DNA, could enable the enhancement of the performance of biosensors by the formation of complementary structures with high affinity. However, Biological recognition elements in electrochemical biosensors offer distinct advantages and encounter specific challenges. Although aptamers exhibit high specificity and affinity, granting versatility in numerous applications. They may degrade over time and necessitate specific storage conditions. Bacteriophages provide specificity in targeting bacterial strains and rapid response times but are confined to bacterial pathogen detection, requiring prior pathogen knowledge.
Antibodies are renowned for their remarkable specificity and sensitivity, enabling the detection of diverse biomolecules and suitability for point-of-care testing. Still, they can lose efficacy under certain conditions and entail production and storage costs. DNA biosensors, often integrated into portable devices, facilitate the precise identification of genetic markers and early disease detection. Nonetheless, they exhibit sensitivity to complex biological samples and mandate skilled operators and equipment. Each recognition element boasts unique strengths and limitations, underscoring the importance of selecting the most appropriate one based on the specific application and requirements (Table 5).
Table 5. Comparison of various bireceptors
Biological Recognition Elements | Advantages | Disadvantages |
Aptamers | – High specificity and affinity for targets -Versatile applications in various fields -Modification techniques for enhancement |
– Potential degradation over time – Requires specific conditions for storage -Limited to certain types of molecules |
Bacteriophage | -Specificity in targeting bacterial strains – Rapid response time for quick results – Applicable in healthcare and food safety |
– Limited to bacterial pathogen detection – Be not able to detect non-bacterial pathogens – Require prior knowledge of the pathogen |
Antibody | – High specificity and sensitivity – Useful for detecting various biomolecules – Portable and suitable for point-of-care testing |
– Limited to antigen-antibody interactions – May lose effectiveness under certain conditions – Costly production and storage |
DNA | – Precise identification of genetic markers – Early detection of diseases – Integration into portable devices |
– Sensitivity to complex biological samples – Requires skilled operators and equipment – Limited to DNA-based diagnostics |
4. The impact of nanomaterials in enhancing the performance of biosensors for Health Monitoring
4.1. Enhancement Sensitivity and Precision
Nanomaterials brought about remarkable improvements in the performance of electrochemical biosensors. One notable advantage is their role in boosting sensitivity and accuracy. Biosensors can detect even the tiniest amounts of target molecules by harnessing nanoscale materials like nanoparticles, nanowires, and nanotubes. This is crucial in medical diagnostics, where early disease detection relies on spotting extremely low biomarker levels. Nanomaterials also increase biosensors’ precision, reducing the chances of incorrect results. Biosensors achieve precise molecular recognition through surface modifications with nanomaterials, ensuring that only the intended biomolecules are identified.
4.2. Rapid Response Times
Nanomaterials significantly reduce the response times of electrochemical biosensors. The substantial surface area relative to their size enables nanomaterials to facilitate rapid electron transfer, resulting in quicker sensor responses. This rapidity is indispensable in point-of-care diagnostics, where swift results are vital for timely decisions. For example, in detecting infectious pathogens or monitoring glucose levels in diabetic patients, biosensors enhanced by nanomaterials can deliver nearly instant readings. This accelerated pace enhances patient care and has far-reaching implications for environmental monitoring and food safety.
4.3. Improvement Stability and Longevity
Nanomaterials play a vital role in raising the stability and durability of electrochemical biosensors. The unique attributes of nanomaterials, including high chemical stability and resistance to degradation, position them as ideal candidates for enhancing sensor longevity. By incorporating nanomaterials into the sensor’s architecture, researchers can ensure that the biosensor maintains its performance over an extended period. This aspect proves especially important for continuous monitoring applications, such as wearable biosensors for health tracking. Additionally, nanoparticles can strengthen biosensors’ resistance to damage so they can work well in various environments, including high and low pH. Because of their flexibility, electrochemical biosensors can be used in various circumstances, making them dependable devices.
5. Identification of Other Analytes
The electrochemical biosensor can detect bacteria and various proteins, including glucose levels, cancer markers, etc.
5.1. Detection Glucose
Han et al. used genetically modified M13 phages. Researchers discuss the synthesis of genetically engineered phage-templated MnO2 nanowires. These nanowires play a crucial role in developing an effective electrochemical glucose biosensor that functions optimally under neutral pH conditions. This research contributes significantly to the field of biosensor technology by introducing a novel approach for glucose detection in a neutral pH environment [9].
The study represents a significant step in plant biology by introducing DNA aptamers as plant biosensors, a previously unexplored area. Their use in plants was limited due to DNA delivery challenges. However, MOU et al. developed a more efficient method than current techniques for inserting DNA into Arabidopsis and tobacco leaf cells. This new method successfully introduced a glucose DNA aptamer sensor into Arabidopsis, enabling glucose detection. This innovation paves the way for using DNA aptamer sensors to study plant biology in-depth, potentially transforming our understanding of plants and their key processes [10].
At UT Dallas, researchers developed a slim, flexible electrode for real-time under-skin glucose monitoring. Easily calibrated in the body, it remains unaffected by other blood substances. Its accuracy is verified against known glucose levels in blood samples [11].
Malmo University, The enzyme on the sensor converts the glucose in the blood and prompts a reaction that the sensor registers [12].
Vallée-Bélisle et al. developed Electrochemical DNA-Based Immunoassay to detect Small Molecules in blood. This research focuses on creating a method for quickly and accurately detecting small molecules in whole blood, which could greatly improve healthcare worldwide. This method, previously tested with DNA strands, uses a process where large antibodies attached to a DNA strand make it harder for that strand to connect with another. Named CeSHHA, this electrochemical test can measure small molecules in complex environments like blood or soil. This affordable testing method has significant potential for on-the-spot diagnosis and monitoring treatments [13].
At Complejo Industrial Chihuahua, researchers developed a new sensor made with laser-induced graphene and silver nanoparticles (AgNPs) to detect glucose levels in saliva. This non-enzymatic sensor provides an innovative way to measure glucose, offering a more straightforward and potentially more reliable alternative to traditional methods [14].
5.2. Identification other protiens
Günter Mayer et al. introduced an innovative aptasensor for detecting lysozyme, utilizing screen-printed electrodes modified with carbon nanotubes. This work represents a significant contribution to biosensors, offering a label-free approach for the specific detection of lysozyme[15].
At the Austerian Institution of Technology (AIT ), researchers developed a cost-effective novel biosensor based on nanomaterial to recognize nucleic acids and proteins in blood or saliva samples [16].
Keck Graduate Institute, Aran et al. designed the portable graphene-based biosensor to identify proteins [17].
At UT Dallas University, Schmidtke et al. designed a novel enzymatic biosensor for quickly detecting acetylcholine, an important brain chemical. This tool uses a unique method, electrochemical impedance spectroscopy, and a specific enzyme, acetylcholinesterase, attached to tiny gold electrodes. This design helps accurately measure acetylcholine in the brain, which is usually challenging because of the brain’s complexity and the short life of acetylcholine. The biosensor works well in different environments, including brain tissue, showing promise for future brain research [18].
At Monteral University, researchers developed DNA-based electrochemical sensors to identify proteins in blood [19].
Institute of Animal Reproduction and Food Research, researchers created new platforms and systems for electrochemical (bio)sensors, focusing on those that incorporate carbon or gold nanomaterials in their transducer elements. They used a variety of biological molecules as receptors, including proteins, enzymes, antibodies, single-stranded nucleic acids (ssDNA), aptamers, and ssDNA G-quadruplexes, aiming to detect biomarkers for diseases like cancer, cardiovascular issues, neurodegenerative conditions, and diabetes. Additionally, they explore how specific food components affect human health, specifically lipoprotein oxidation and protein aggregation, and examine new synthetic compounds that can inhibit critical proteins in the body. Their work also extended to developing systems that can deliver therapeutic compounds directly to cancer cells in a targeted and controlled manner [20].
6. Limitation and future perspective of Electrochemical biosensors in point-of-care diagnostics
Electrochemical biosensors, while promising, have several weaknesses and limitations. Firstly, they often require complex instrumentation and skilled operators, limiting their accessibility in resource-constrained settings. This complexity can result in higher costs, challenging widespread adoption, particularly in low-income regions. Additionally, electrochemical biosensors may face difficulties in detecting multiple targets simultaneously, a crucial requirement for comprehensive diagnostics in certain situations. These limitations underscore the need for user-friendly and affordable biosensors to perform multiplexed assays.
Another limitation is the potential for interference from complex biological matrices. Biological samples often contain various substances that can interfere with the electrochemical signals, leading to false results. Addressing this challenge requires advanced surface modifications and signal processing techniques to enhance specificity and reduce cross-reactivity. Furthermore, the stability and durability of electrochemical biosensors can be compromised over time, affecting their long-term reliability. Researchers are actively working on improving sensor materials and coatings to mitigate these issues and extend the biosensors’ lifespan.
In terms of future prospects, electrochemical biosensors hold immense potential. Miniaturization and the integration of nanomaterials offer exciting avenues for improving sensitivity, reducing response times, and increasing the robustness of these sensors. Advances in artificial intelligence and machine learning can further enhance data analysis and interpretation, making biosensors more intelligent and capable of delivering actionable insights. Additionally, the integration of biosensors into wearable devices and the Internet of Things (IoT) ecosystem opens up new opportunities for continuous health monitoring and real-time data transmission. These developments indicate a bright future for electrochemical biosensors in revolutionizing diagnostics, disease management, and personalized medicine.
7. References
[1] Wu, J., Liu, H., Chen, W. et al. (2023). Device integration of electrochemical biosensors. NatureReviews Bioengineering, 1, 346-360. DOI: 10.1038/s44222-023-00032-w [2] https://www.creative-biolabs.com/ [3] Pankratov, D., Bendixen, M., Shipovskov, S., Gosewinkel, U. and Ferapontova, E.E., 2020. Cellulase-linked immunomagnetic microbial assay on electrodes: Specific and sensitive detection of a single bacterial cell. Analytical Chemistry, 92(18), pp.12451-12459. DOI: 10.1021/acs.analchem.0c02262 [4] https://cff.dcci.unipi.it/latest/cff2023.html [5] https://iem.creative-biostructure.com [6] http://www.societasim.it [7] Moghtader, F., Congur, G., Zareie, H.M., Erdem, A. and Piskin, E., 2016. Impedimetric detection of pathogenic bacteria with bacteriophages using gold nanorod deposited graphite electrodes. RSC advances, 6(100), pp.97832-97839. DOI: doi.org/10.1039/C6RA18884B [8] https://www.superbioz.com/rapid-detection-of-superbugs-using-dna-nanosensor.html [9] Han, L.; Shao, C.; Liang, B.; Liu, A. Genetically Engineered Phage-Templated MnO2 Nanowires: Synthesis and Their Application in Electrochemical Glucose Biosensor Operated at Neutral pH Condition. ACS Appl. Mater. Interfaces 2016, 8, 13768–13776. DOI: doi.org/10.1021/acsami.6b03266 [10] Mou, Q., Xue, X., Ma, Y., Banik, M., Garcia, V., Guo, W., Wang, J., Song, T., Chen, L.Q. and Lu, Y., 2022. Efficient delivery of a DNA aptamer-based biosensor into plant cells for glucose sensing through thiol-mediated uptake. Science Advances, 8(26), p.eabo0902. DOI: 10.1126/sciadv.abo0902 [11] https://be.utdallas.edu/ [12] https://mau.se/en/news/new-smart-solutions-could-replace-current-infection-testing/ [13] Mahshid, S.S., Ricci, F., Kelley, S.O. and Vallée-Bélisle, A., 2017. Electrochemical DNA-based immunoassay that employs steric hindrance to detect small molecules directly in whole blood. ACS sensors, 2(6), pp.718-723. DOI: 10.1021/acssensors.7b00176 [14] Aparicio-Martínez, E.P., Vega-Rios, A., Osuna, V. and Dominguez, R.B., 2023. Salivary Glucose Detection with Laser-Induced Graphene/AgNPs Non-Enzymatic Sensor. Biosensors, 13(2), p.207.
DOI: 10.3390/bios13020207 [15] Rohrbach, F., Karadeniz, H., Erdem, A., Famulok, M. and Mayer, G., 2012. Label-free impedimetric aptasensor for lysozyme detection based on carbon nanotube-modified screen-printed electrodes. Analytical Biochemistry, 421(2), pp.454-459. DOI: 10.1016/j.ab.2011.11.034 [16] https://www.ait.ac.at/ [17] https://www.kgi.edu/ [18] https://be.utdallas.edu/people/faculty/david-schmidtke/ [19] Mahshid, S.S., Camiré, S., Ricci, F. and Vallée-Bélisle, A., 2015. A highly selective electrochemical DNA-based sensor that employs steric hindrance effects to detect proteins directly in whole blood. Journal of the American Chemical Society, 137(50), pp.15596-15599. DOI: 10.1021/jacs.5b04942 [20] https://pan.olsztyn.pl/research-dissemination/scientific-groups/biosensors/